4 Comments / Projects / By PonoBill
I’ve had a few questions about how and why I’m doing a solar/battery system for Fritz, what I’m using and how I chose it. This is going to be a fairly long post with a bit of math, so if you’re interested, get a cup of coffee and settle in.
Fritz is an extreme experiment. If I can make this system, sized in this way, work for Fritz, then it can be done almost anywhere. Fritz is a 1978 GMC motor coach. It’s not designed for this kind of stuff in ANY way. I’m using this coach because I appreciate the size, build quality, brilliant design, and overall character of these coaches. They’ve never been equaled. But it’s an insane platform for this experiment–which makes it perfect.
RV appliance and comfort systems haven’t changed much since the 70’s with pretty good reason: The systems work. A quick review will help the inexperienced, you veteran RVers can skip a few paragraphs.
A typical RV has a refrigerator, stove, a water heater, a furnace, and an air conditioner with propane tanks and a generator to make everything work when shore power isn’t available. The water heater is often dual-mode, gas/electric, as is the refrigerator. The furnace and stove burn propane, and the air conditioner is purely electric and may have an electric heating element to do double duty for space heating. With a few minor exceptions, all the electric components use standard 115V alternating current, just like your house.
In general, this system works fine, and many people are satisfied with it. There are some inconveniences and a few dangerous aspects, but it works. It’s good. But it’s not great, and it’s possible to do much better. Let’s start with propane. If it hadn’t been used for the last 50 years, with well-established appliances readily available, there is simply no way it would be introduced as the ideal fuel for appliances in confined spaces. It’s acceptable because it’s deployed and readily available. Lots of energy in those bottles, readily available–but also toxic and explosive. You’d have to be a little nutty to go ripping out all your propane-fueled appliances just because they might kill you, and that’s not really why I did it with Fritz, but yeah, I’m that kind of nut.
And then there are generators–gas or diesel. Generators suck. End of discussion. If you didn’t need one you’d never have it. They’re noisy, they stink, and they use a lot of fuel. It’s feasible to operate without one today, even if you’re boondocking. So that’s what I intend to do.
Appliances
I think the future for RV’s is all-electric, even the drivetrain, but it will take quite some time to get there. To my knowledge, there is one low voltage compressor-type refrigerator available for RV’s, the Nova Kool refrigerators from Canada. They represent a tiny fraction of the number of adsorption-type refrigerators sold today for RV use. They are ideal for all-electric use, drawing 2.6 amps at 24vdc.
There are no commercial alternatives to propane stoves, so that little carbon monoxide headache you get after cooking dinner is likely to persist a while. I’m replacing mine with induction plates–portable, efficient, versatile and cheap. Mine can be controlled from a smartphone and serves as a slow cooker and sous vide systems with temperature control of the contents of your pot via an attached thermometer. They draw 1600 watts at 115V. We also installed a Breville Smart toaster/oven that serves well for baking. At 1800 watts (80 amps at 24VDC) it’s a nasty load. My system can accommodate it, though we’re unlikely to do much baking without shore power.
The biggest issue most people question is the air conditioner–and rightly so. AC is a near requirement for the small space of an RV, and it’s a consistent load. You can decide not to bake a pie because you don’t want to use up your battery reserve, but who wants to swelter? RV air conditioners are 115VAC systems–no one has seen fit to build a DC one yet. It’s clearly feasible, but there isn’t a market demand for it. So my system needs to accommodate that AC load. A typical Coleman Mach8 heat pump draws 16 amps at 115VAC, or 1840 watts. Ignoring small inverter efficiency losses that means 76 amps at 24VDC. Starting current is around 60 amps at 115V, so 6900 watts or 290 amps at 24VDC. Yikes. Of course, a soft-start capacitor will help with that, but I’m sizing my inverter and system to accommodate that very hefty momentary load. More on that later.
Water heating is pretty straightforward. It’s not a continuous load and the water heaters in RVs are small and reasonably efficient as long as you don’t try to use much hot water. I’m simply not going to use the gas side–electric only. Mine takes 1400 watts. Another hefty load, but you can decide when and how to use it, and even if you just leave it on all the time, the load is only what is required to make up for hot water use and heat loss. I’ve taken advantage of eliminating the gas and required venting to substantially improve the insulation.
For heat, I’m using the AC heat pump, and I’ve added an electric strip for those occasions where the outside air temperature is too low for the heat pump to work. I’m a warm weather type, I don’t think Fritz is going skiing so I don’t think this is a big concern. I could be wrong.
Summing up the appliance loads:
[table id=2 /]
Turn everything on at once and it’s about 280 amps at 24V, which is 6686 watts. That’s why I installed an AIMS 6000 Watt low-frequency pure sine inverter that can handle peak loads to 18,000 watts for up to 20 seconds (no, I’m NOT going to test that claim), and why my battery to inverter circuit is fused for 300 Amps. And obviously, this system is going to depend on a lot of solar power, and some big lithium batteries. I could do this with AGM batteries, but who would want to? Lithium batteries will last much longer with these loads, are much lighter, and are ultimately less expensive. There are some legitimate concerns about Lithium batteries, especially for those who live in the frozen north, and we’ll talk about those later.
Anyone undertaking a similar project, even one of more modest ambition, can make the same kind of calculation. Decide what the minimum size inverter should be by adding up all the loads that could be put on it. You can choose to limit the loads by having electrical distribution sub-panels that provide inverter power only to those loads you choose, or you can go for broke and size your inverter to supply everything, worst case, as I have. Big low-frequency inverters aren’t cheap, but they aren’t the monster expense they used to be. My 6000-watt inverter was about $1300. That’s for a highly-rated inverter charger that supports multiple battery chemistries, provides automatic power transfer with a 10ms switching time, hibernates at 25 watts, and has built-in GFCI and battery prioritization. In other words, a top of the line, feature rich inverter that could provide uninterruptible power to a small house. That might not seem all that exciting to most folks, but it sets my geeky heart fluttering.
The calculations for solar panel requirements, controller size and type, and battery bank require a little more work. We’ll get to that.
Solar Stuff
The underlying rap about solar power is that it’s expensive. People still apparently think we live in 1985. The current cost per kilowatt for solar panels is about $1.00 per watt, and if you don’t simply buy panels from the handiest source they are less. I paid $126 each for nine 310 watt panels. That’s $.40 per watt. Six of the panels are going on Fritz and three will go on my Airstream race car hauler–eventually. These big panels are not typically used in RV installations–the 100-watt panels are easier to handle and install–but I’m doing something a bit different anyway, I’m not installing a few hundred watts of solar, my Fritz installation is 1860 watts. You can get good quality 100 watt panels for about $100, which is $1 per watt, and that’s a pretty remarkable price. I have no trouble remembering when panels were $20 per watt–I was working at nuclear power plants at the time and people were saying solar power was the future. I thought they were morons. Oops.
If you’re trying to do a simple installation, these are not the panels for you. They require substantial fabrication to install.
Most modern Class A RV’s have a lot more roof than a 1978 GMC motorhome. It’s feasible to do a flat installation of several thousand watts without getting too creative. And on those big flat roofs, you could avoid shading just with positioning. You could also install a tilting system to improve the angle of the sun’s rays and substantially increase panel output when the sun is not overhead or in winter months. For Fritz, I simply raised the entire installation above all the roof-mounted equipment and covered the entire roof. I could make some of the panels tilt, but the improvement in low sun angle performance would come at a cost in complexity that would only work with the rig pointed in particular directions. If I find it’s necessary, I can retrofit tilting mechanisms, but for now, I just settle for a lot of cells.
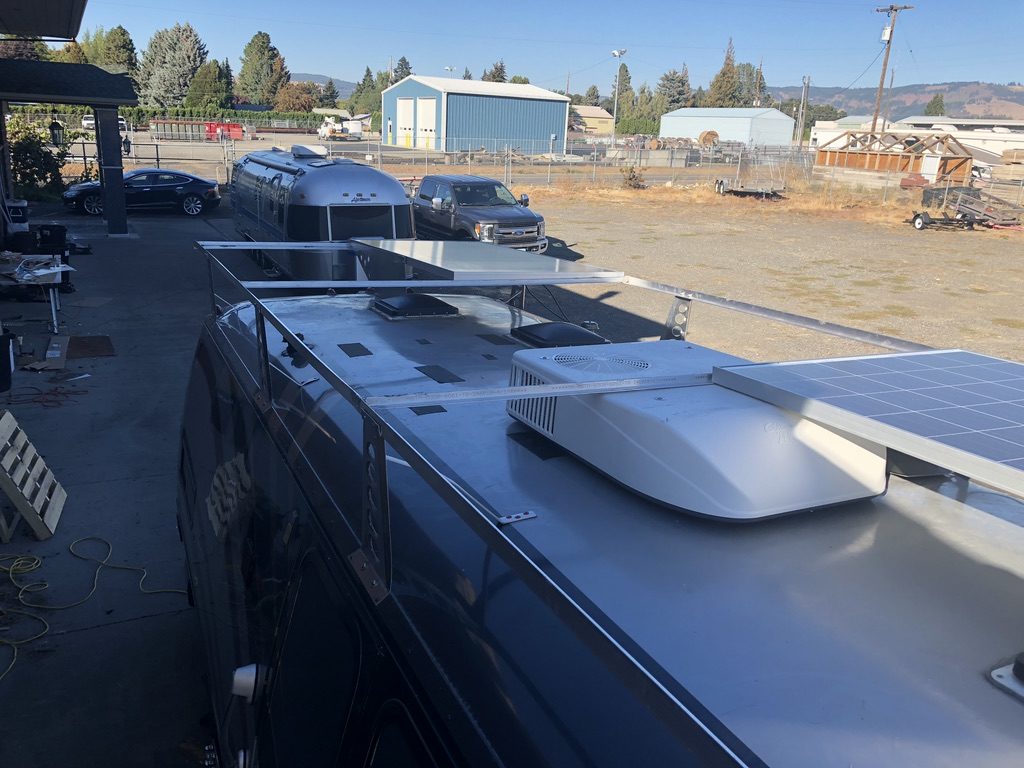

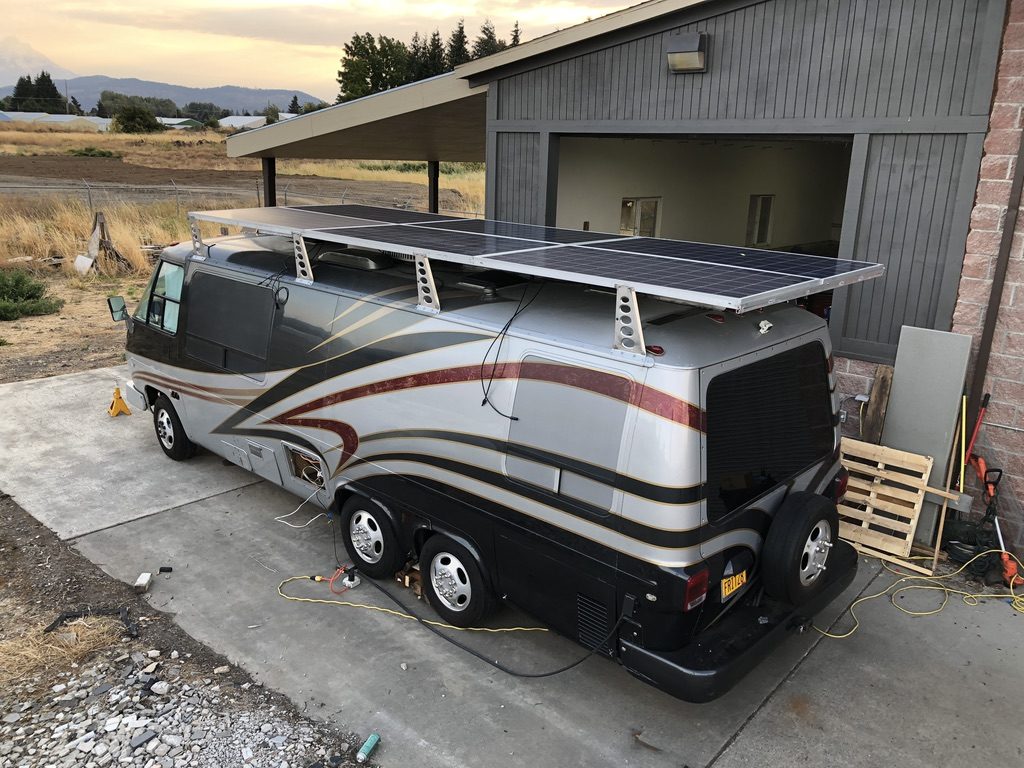
If you’re doing an installation directly on your roof you need to map out how the equipment will shade potential locations for installation. Based on that map, you’re not only going to need to make decisions about which panels sometimes get shaded, but also which to connect in series or parallel. If one panel in a series string gets shading you lose most of the output of the entire string. That doesn’t harm anything, but it means a little shade takes a big bite out of your charging rate. There are good reasons to connect some of your panels in series, and yes, we’ll get to that soon. But if you have two panels in series, and one is shaded, neither will do you much good. If they’re in parallel and one is shaded, the other will still deliver power.
Charge Controllers
Here’s an easy one–get an MMPT controller. If you don’t want to know why, you just want to know how to select the right size, then skip the next few paragraphs.
Back in the old days of solar a typical panel generated about 16 to 18 volts and not a lot of amps. You could connect them right to a battery and just charge it. With typical use, the battery would never overcharge. If you didn’t draw enough power off, the system would overcharge the battery and slowly boil the battery dry. The earliest controllers simply opened the charging circuit when the battery was fully charged. PWM (Pulse width modulated) charge controllers do this a bit more gently, changing the amount of time the solar panels are connected to the battery. If the battery voltage is low the connect time is long. When the battery voltage gets higher the connected “pulse width” gets narrower, until it stops charging the battery almost completely. The voltage coming out of the PWM controller doesn’t change–just the amount of time the panel is connected. That’s fine for panels with lower cell counts, but today’s 60, 72 and 90 cell panels put out much higher voltages. The controller will be effectively averaging the output voltage of perhaps a 40 volt set of panels to something around 14 volts, so you’ll lose about 50 percent of the panel output. Of course, no one actually does that, most PWM installations match the panel voltage as close as possible to the battery charging voltage, but that means heavier wiring and more resistive losses for a similar system with an MMPT controller. The typical efficiency increase of an MPPT controller over PWM in properly sized systems is likely in the 30 percent range, but that’s still too much to give up.
MPPT (Maximum Power Point Tracking) controllers are dc to dc converters that convert the panel voltage to the optimum charging voltage of the battery. The panels can operate at the most efficient voltage while the output voltage of the controller stays relatively constant, delivering as many amps as the solar cells can produce. The most sophisticated controllers can accommodate multiple battery chemistries, charging profiles, and output voltages. You can also use series connected cells, which means you can use lighter wire and suffer less energy loss in the wiring from the solar panels to the controller and from controller to batteries. The electronics are more sophisticated, so MPPT controllers are a little more expensive, but that’s changing as the MMPT controllers become the standard and are produced in larger quantities. MPPT will completely replace PWM in the near term.
Choosing and Sizing
All you need to do is pick the right controller. Yes, you can buy a properly sized kit, but you should know how to figure out something this simple. MPPT controllers are sized by output current. A 60 amp controller handles 60 amps of output, regardless of whether the output voltage is 12, 24, or 48 V. If you choose to have a 12 V system output and you have 720 watts of solar panels you need a 60 amp controller (720watts/12volts=60amps). If you have 24 V system output and have 720 watts of output you need a 30 amp controller (720watts/24V=30amps) and obviously, for a 48V battery at 720 watts you could use a 15 amp controller.
You also need to pay some attention to the input voltage. For an MPPT controller to be happy, the output voltage of the solar panels should be substantially more than the battery voltage, but not so high that the system can’t handle the voltage conversion. That’s a highly variable number that the manufacturer will supply, but a decent starting point is at least double the battery voltage and not much more than four times the battery voltage.
In Fritz, I’m using two MakeSkyBlue 60 amp MMPT controllers. I have six panels, connected in series pairs. The open circuit voltage of my 72 cell, 310-watt panels is 40V, so each series pair puts out 80V and about 7.75 amps. Typical practice is to connect rooftop panels into a combiner box which usually contains fuzes on the positive lead of each panel set and then combines the leads into a bus for the positive and negative sides. These combined connections are then lead into the cabin and to the controller through a single lead each for the plus and minus connection. I hope that makes sense, it was a little torturous to write.
I’m not doing that. I used inline fuzes that connect to the positive MC4 plugs and I ran all six panel connections–three positive, three negative–down to the two controllers.

Each controller has two sets of panel connectors, so two sets of connectors go to one controller and one set go to the other. Doing it that way gives me more options for output voltages and battery chemistry. The capacity of a single 60 amp controller was in the ballpark for connecting all three sets of panels to a single controller, but I would have been pushing the capacity limit. The controllers are about $150. For that price, having some redundancy, more flexibility, and more capacity margin seems like a bargain.

Well, OK, we’ve got solar power flowing to the controllers. This is getting ridiculously long. Let’s end here for now and I’ll do the rest in a few more posts.