My recent video, “Solar Grounding and Bonding Demystified”, caused quite a stir. Will Prowse called it an awesome video, but lots of other folks commented that I didn’t know what I was talking about. It’s a controversial issue, and some folks think that if experts don’t agree on the solutions that they don’t need to worry about it. They might get away with that, or they might wind up with thousands of dollars worth of slagged junk. If you live in the wrong place, the likelihood of toasting your gear goes from near zero, to almost a certainty without proper grounding. Because we’re not talking about direct strikes on your panels. That’s extremely unlikely and if it happens your equipment is gone, no matter what you did to prevent it (short of building a Faraday cage around your home). The lightning lottery is mostly about nearby strikes, and if you haven’t grounded your system properly one good local storm can destroy all your gear–even if you have it festooned with SPDs. Some SPDs are valuable protection, some are worthless, but even the good are only as good as the available earth ground and need to be installed carefully to really protect your gear. We’ll cover how briefly.

This video is NOT about bonding and grounding to manage system faults. And it’s not about direct strikes. It’s about dealing with the far more likely induced high voltage spikes from nearby strikes. And by “nearby” I mean as much as a few miles away in some cases, though the closer a bolt strikes, the more likely you’ll have damage. Even cloud-to-cloud lightning five or more miles away can be surprisingly damaging. Because lightning produces a pulse with a very steep, short rise time, and a crazy amount of power, which gives it the characteristics of radio frequency AC. And we all know that can travel distances and induce currents in other conductors. It’s hard to stop, and hard to manage. The best way to get rid of it is to let it return directly to the source–the earth–on the shortest path that doesn’t include us, our expensive equipment, or our home.
The likelihood of nearby strikes is largely a function of where you live, and just because your state does well in the lightning lottery doesn’t mean you’re home free. Specific locations vary widely, and the problem is not just lightning. Dry, windy places build lots of static charge. Not as dramatic as a lightning strike, but still potentially harmful to equipment. But yes, if you live in the hot zones, you REALLY need to understand the risk and how to mitigate it.
And while places like Colorado might seem to do well in the lightning lottery, places like Denver most assuredly do not. Here’s the strike map for the Denver area. Note that while most of the state has a low density of strikes, and some other areas have a sea of green, there are small areas that have high activity. If you really want to know what your risk of damage is, you need to search out local information. Though my latest installation is in the relatively inactive Pacific Northwest, I looked at local activity and decided I need to make my grounding system as robust as I can.

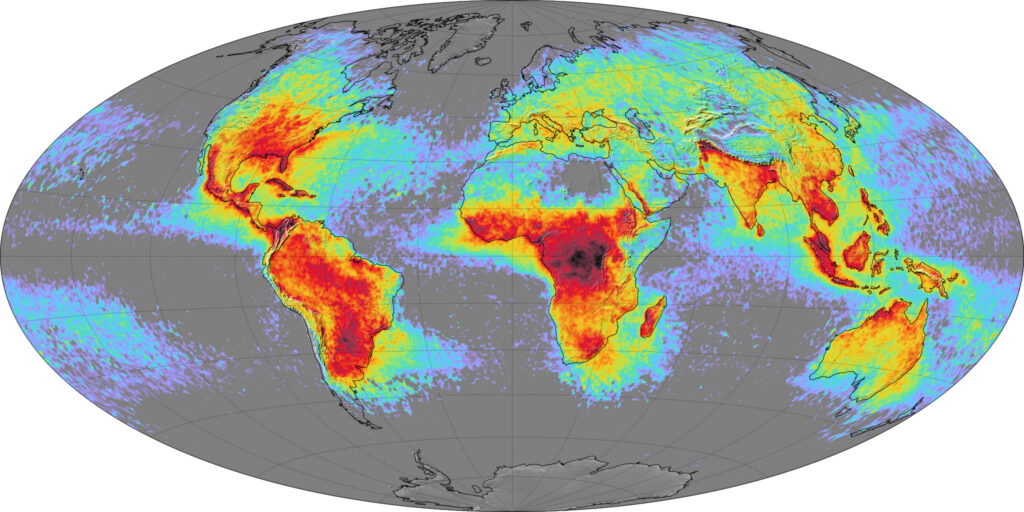
Youtube has lots of videos about grounding PV systems to mitigate induced voltages from atmospheric phenomena. Lots of them are just flat out wrong (in my not so humble opinion), some seem well researched, but none are as clear and authoritative as this video “How to ensure safe and effective solar grounding”. I especially like it because it supports what I think is the right approach. Yes, I’m human, I’m always willing to change my mind if I see solid evidence I’m wrong, but it’s nice to find videos that agree with me. The presenter quotes NEC and NFPA codes like he wrote them. Of course he’s reading from a teleprompter, but he did the research. Finding specific information in the NFPA is not easy. There is real expertise in how to protect your gear from voltage spikes–from the engineers that need to deal with it almost daily. If you’re protecting radio gear connected to a tower that sticks up hundreds of feet in the air, or a transmission line that stretches for hundreds of miles, you get whacked frequently. The approach in the schematic above is the bare bones of how they do that. Their earth grounds are far more elaborate than an eight foot rod pounded in the ground, but the underlying principle is exactly the same. If you’d like to see how extreme that gets, thumb through the 110 pages of NFPA 780. And realize that what you see is the legally required minimum, not the outer edges necessary to adsorb strikes and still operate without a glitch.

The schematic above is the grounding approach I believe is the best combination of local earth grounding with one or more grounding electrodes connected to the frames and mounting structure for the PV array with short, straight runs of wire to keep voltage induced in these structures from a nearby lightning strike from entering the inverter and related equipment by conducting the voltage to ground. The biggest objection to auxiliary earth grounding is that multiple separated earth grounds can have a voltage induced between them by a nearby strike. This design prevents that in two ways. The frame and mounting structure ground is connected to the system ground in the inverter which provides a low resistance connection to the service entrance grounding electrode. In addition, a bonding conductor is attached to both grounding electrodes under grade. The conductor, in my case, is bare 6AWG copper wire in the trench that I needed to dig anyway to carry the AC line from my inverters and batteries (located in my container) to the load panel. The red arrows depict SPDs that conduct surge voltage in the DC lines leading to the inverter to the earth ground, and and SPD that protects the output of the inverter
I recognize that there is good reason to say that system grounds already provide a path to keep the potential on the frames and mounts close to the same as the service entrance. You certainly can and should tie a system ground wire to the structure of the array as the schematic shows. The path is through your equipment and your home, but code says it’s OK, and many people do that. It’s probably adequate. Probably. But that’s a somewhat unreliable connection, relying on a chain of fairly funky screw connections and runs of wire with multiple bends and kinks. I don’t see why you’d want to be leading induced current from lightning, EMP pulses, solar flare spikes, or static into your equipment without ensuring that there is a very low resistance, dedicated path to ground to reduce the difference in potentials to a low value.
The NEC codes go back and fourth on this. The 2014 edition says remote equipment (unattached structures) MUST have an earth ground. Later editions say they MAY have, but that’s because most of the requirements regarding lightning and static dissipation have been moved to National Fire Protection Association (NFPA) 780. NEC 250.4 says the unattached equipment needs to be earth grounded and then sends the reader off to NFPA 780-2020 for details. The 2020 version of the 780 is only 110 pages long but it is unbelievably dense and I haven’t found any useful way to parse out the requirements for solar installations. Basically every conceivable way to deal with direct and nearby lightning strikes are covered–exhaustively–including the above method with local earth grounds, bound together below grade. That leads me to the belt and suspenders conclusion that a local earth ground (perfectly OK under code) bound (connected) to the service entrance earth ground below grade is a good solution. No, it isn’t an explicit code requirement, just (I think) a best practice. It does add cost, but if you’re already trenching to connect your equipment to the service entrance then it’s ground rods and some bare #6 wire. Maybe $200 if the run isn’t super long, and if it is long, then I’d say doing so raises way above just best practice.
I still plan to add SPDs as shown in the schematic. They seem redundant once everything is well grounded but they also protect against line voltage spikes, which are common. The performance of SPDs, and their effective life should be substantially extended with this grounding approach. Most good SPDs use Metal Oxide Varistors (MOVs) or gas discharge tubes which work fast and well, and reset automatically. But they can degrade with each substantial power spike they block. In the case of a nearby lightning strike they are probably as fried as the rest of your equipment if you don’t have grounding that reduces the induced voltage on the structure and frames of your PV array and binding between the ground rods to minimize the differential potential between them. Don’t think of SPDs as lightning protection, they really aren’t, though the source of power spikes could well be distant lightning strike. The spike can also be from line faults, switching operations, or large machinery cycling. Good quality SPDs are not cheap, and cheap ones are generally worthless. MOVs have several failure modes, including fail open, fail shorted, or fail to a linear resistance. Fail open doesn’t represent an issue other than the need to replace the SPD, but fail shorted or fail linear resistance causes fault currents that mean the protected equipment usually can’t be used until the SPD is removed–unless the SPD has internal fuses which remove it for you–some do not.
This seems like a good time to talk about SPD installation. They need to be installed like the conductors to an earth ground, because that what they are. Short straight runs of wire with minimal bends. No service loops or excess wire. They need to be installed on the supply side of equipment, which means on the top of any panel or box, where the supply enters. They don’t do much good if they clamp and divert the spike after the connection to the equipment. We’re not dealing with low frequency AC or DC. If there’s a parallel path through your equipment the spike will appear there too.
The SPD has to be connected to a system ground leading to a high quality earth ground. If there isn’t a direct path to a good earth ground the SPD is almost worthless.
You might need a SPD on both the input and output side of the equipment you want to protect. Remember that current that can flow through your equipment from the ground rods if you don’t have them all bound together. The direction of current flow is from the highest potential rod to the lowest, and that depends on the direction the spike is being propagated from. Which direction is that? No idea, it’s random.
By now you probably see why the grounding method in the schematic above is the best practice for protecting your gear from induced spikes.